Danny Van Quaethem has a master’s degree in Germanic Philology (English-German) at RU Gent. Then he obtained the diploma of financial analyst (ABAF). He wrote for ten years for investment magazines (7 years for Afinas Report and 3 years for De Belegger). In 1997 he started at Société Générale Private Banking Belgium (formerly Bank De Maertelaere). He worked exclusively as a financial analyst covering equity markets, focusing on a number of sectors (pharmaceuticals, chemicals, consumer goods). At Econopolis, Danny is Senior Equity Analyst.
Radiopharmaceuticals: A Panoramic View
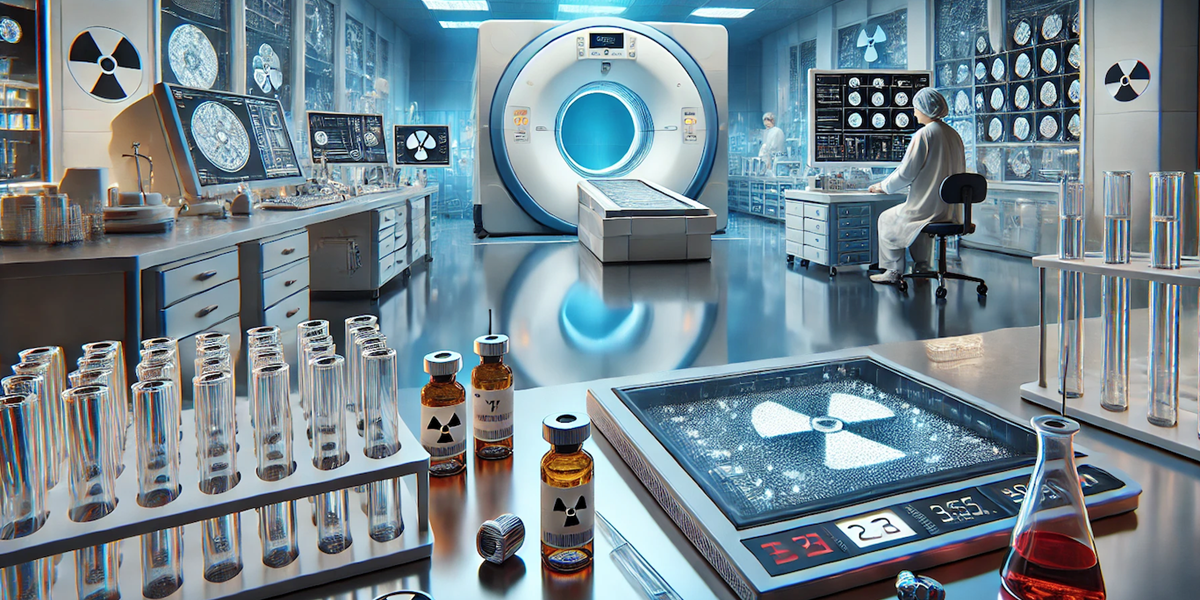
This Long Read delves into the therapeutic potential, market growth, and current developments surrounding radiopharmaceuticals—a sector poised for significant impact in the coming years. Radiopharmaceuticals, also known as radioligands, represent a highly promising new approach to cancer treatment. These compounds consist of a radioactive isotope linked to a pharmaceutical agent, such as an antibody, antibody cocktail, or small molecule, enabling targeted delivery of radiation to specific tissues or organs.
Radiopharmaceuticals sit at the intersection of medicine and nuclear science. They contain radioactive isotopes, known as radionuclides, that emit radiation in the form of alpha particles, beta particles, or gamma rays. When administered to a patient, radiopharmaceuticals can serve both diagnostic and therapeutic purposes.
Diagnostic Applications
In diagnostic imaging, radiopharmaceuticals are used to visualize organs and tissues within the body. The radionuclide in the radiopharmaceutical emits radiation that is detected by specialized imaging equipment, such as a gamma camera or PET scanner, allowing doctors to identify abnormalities that may indicate disease.
One common application is nuclear medicine imaging. A radiopharmaceutical is injected into the patient's bloodstream and circulates throughout the body, accumulating in specific organs or tissues based on its chemical properties. Once it reaches its target, images are acquired to visualize the distribution of the radionuclide.
Another important application is positron emission tomography (PET). In PET imaging, a radiopharmaceutical emitting positrons is administered. When a positron encounters an electron, they annihilate (a process where both particles are destroyed), producing two gamma rays that travel in opposite directions. These gamma rays are detected by a PET scanner to create 3D images of the body. PET is particularly useful for imaging metabolic activity, making it valuable for diagnosing and staging cancer, as well as evaluating brain function and other neurological disorders.
Therapeutic Applications
Therapeutic applications use radionuclides that emit high-energy radiation to destroy cancer cells. A radiopharmaceutical is administered to the patient, where it accumulates in tumor cells. The radionuclide then emits radiation that damages the DNA of the cancer cells, ultimately leading to their death. Radiation therapy can treat a variety of aggressive and invasive cancers, including prostate, pancreatic, bladder, lung, glioblastoma, ovarian, and blood cancers like lymphoma and leukemia.
Another approach is targeted radionuclide therapy (TRT). In TRT, a radiopharmaceutical is designed to target specific molecules on the surface of cancer cells, delivering radiation directly to the tumor while minimizing damage to healthy tissues. TRT has shown promise in treating neuroendocrine tumors and prostate cancer.
Therapeutic advantages of radiopharmaceuticals
The goal is to select the isotope that best aligns with the target biology and indication, potentially broadening the use of radiopharmaceuticals for diagnosing and treating cancer. These drugs offer several advantages: their small size allows for rapid tissue penetration, and tunable pharmacokinetics (how the body processes substances over time) enable target optimization. Additionally, their high affinity and selectivity allow for precise targeting and tumor retention.
These characteristics may result in greater tolerability, improving adherence to optimized dosage regimens. They could also lead to longer, deeper, and broader responses, enhancing patient outcomes. Radiopharmaceuticals show promise in treating microscopic and disseminated cancers, which are among the most difficult to treat. It is known that circulating tumor cells (CTCs) play a crucial role in spreading dormant but lethal cancer.
These developments could potentially transform treatment paradigms, supporting the trend towards personalized medicine. According to Precedence Research, the global radiopharmaceuticals market was valued at around USD 6 billion in 2023 and is projected to exceed USD 13.5 billion by 2033, with a compound annual growth rate (CAGR) of 8.2% from 2024 to 2033. However, other analysts estimate much higher figures. It's no surprise that radiopharmaceuticals are one of the hottest fields in the industry, attracting significant investment from big pharma. Eli Lilly, Bristol Myers Squibb, and AstraZeneca have collectively spent around USD 8 billion on biotech companies developing lead drugs in clinical trials using actinium-225. Bayer and Novartis (marketed drugs: Lutathera for pancreatic cancer and Pluvicto for prostate cancer) already have approved radiopharmaceuticals using different isotopes.
In 2023, Eli Lilly acquired Point Biopharma, a biotech specializing in radiopharmaceutical drugs for prostate cancer, for USD 1.4 billion. In February 2024, Bristol Myers Squibb purchased RayzeBio for USD 4.1 billion. In June 2024, AstraZeneca acquired Fusion Pharmaceuticals, which specializes in next-generation radioconjugates (RCs) for cancer treatment, for USD 2.4 billion. Sanofi recently signed a USD 110 million licensing deal with Texas-based biotech RadioMedix.
Hurdles: trained medical personnel and supply chain
There are still many hurdles to overcome. While substantial clinical research is ongoing, it does not guarantee successful outcomes. Even after FDA approval, numerous challenges remain. As in many industries, finding the right medically trained personnel is a significant issue. Additionally, supply may be insufficient or unsuitable, especially considering the half-life of the isotopes. After a patient is approved for radiotherapy, supply chain limitations can force them to wait more than a month for treatment to be manufactured and delivered to the hospital. Novartis faced supply constraints for Pluvicto, and RayzeBio, now owned by Bristol Myers Squibb, had to pause recruitment for the international arm of one of its radiopharmaceutical drugs for several months this year due to a lack of actinium-225.
To address these challenges, big pharma companies have signed supply agreements with contract manufacturing organizations. Some, like Lilly and Novartis, have also brought parts of the production in-house. Lilly has additionally invested in isotope supplier Ionetix, which operates a facility capable of producing actinium-225, an isotope crucial to many radiopharmaceutical drugs that is in very limited supply. Since actinium-225 does not occur naturally, only about two curies—a measure of nuclear energy—of the isotope are produced each year, enough to treat at most about 2,000 patients. In Belgium, IBA (Ion Beam Applications) owns 31.3% of Pantera, which focuses on the production of actinium-225.
There are many types of radioisotopes, including Technetium-99, Fluorine-18, Lutetium-177, Yttrium-90, Gallium-68, Gallium-67, Rubidium-82, Iodine-123, Iodine-125, Iodine-131, Indium-111, and many others. Actinium-225 offers particular therapeutic potential.
PanTera recently raised sufficient funding to establish a state-of-the-art production facility for actinium-225 (Ac-225) in Mol, Belgium. Its unique production process, employing the photonuclear or 'gamma' route, transforms Radium-226 (Ra-226) into Radium-225 (Ra-225), which subsequently decays into Ac-225. This high-purity Ac-225 is then supplied as an active pharmaceutical ingredient (API) to the global pharmaceutical market. In addition to ensuring quality, PanTera prioritizes sustainability, recycling and reusing the radium in its process. PanTera is already expected to start delivering small quantities of Ac-225 through its American partner TerraPower, founded by Bill Gates. Production at its own facility in Mol is expected to come online around 2028, aligning with a potential commercial breakthrough for these therapies.
PanTera’s process and infrastructure are designed to enable the annual production of more than 100 curies (Ci) of clinical-grade Ac-225 by 2029, allowing treatment for over 100,000 patients per year. The combination of SCK CEN’s unmatched large stock of pure Ra-226 and IBA’s Rhodotron® electron accelerator positions PanTera to become a highly successful and reliable producer of this key medical radioisotope, which is crucial for personalized nuclear medicine.